How Proteins Interact May Hold Key to Future Autism Treatments
UCSF researchers reveal how some gene mutations lead to autism spectrum disorder.
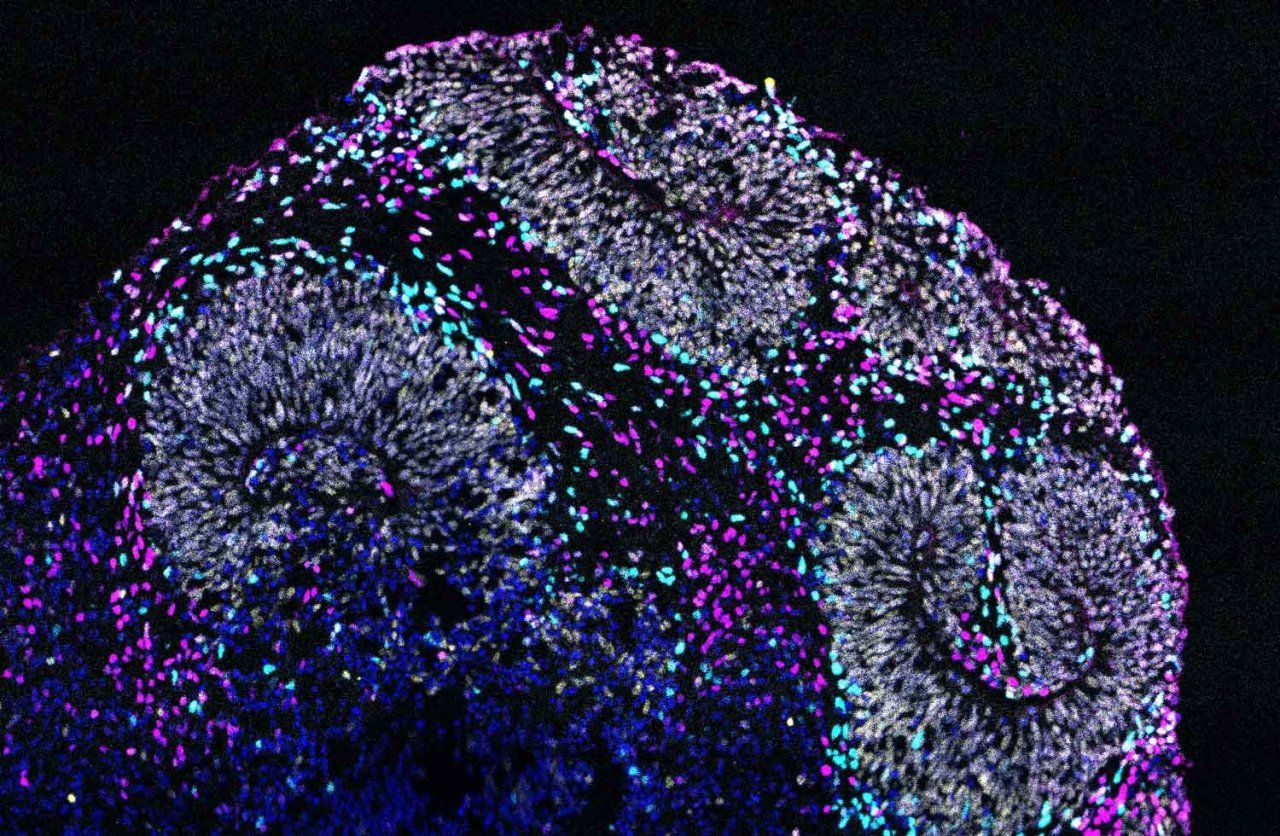
Tremendous progress has been made over the last decade in identifying genes leading to autism spectrum disorders (ASD), especially for those who are severely affected. To date, however, these genetic insights have not translated into improvements in clinical care, particularly for those who need it most.
A cross-disciplinary collaboration at UC San Francisco has taken a new approach that has begun revealing, in detail, how a set of around 100 ASD genes may lead to serious developmental problems, often resulting in severe and profound autism that impacts as many as 20% to 30% of people on the spectrum. This subset of individuals with ASD also often have associated conditions that can greatly influence their quality of life, such as epilepsy, delayed motor development and intellectual disability.
The technique involves looking beyond genes and their mutations, to the proteins they code for. Proteins carry out nearly all the functions in the body, by interacting with each other in a sort of dance. When the proteins are mutated, that dance can involve missteps, or not happen at all, leading to disease.
The protein-centered approach was developed by Nevan Krogan, PhD, director of the Quantitative Biosciences Institute (QBI), who has used it to help identify potential new drugs for cancer and COVID-19. Expanding into the psychiatric realm, Krogan partnered with Jeremy Willsey, PhD, then an associate professor in the Department of Psychiatry and Behavioral Sciences, now adjunct, and Matthew State, MD, PhD, department chair.
We asked the three researchers to explain what they’ve learned so far. They caution that there’s much more work to be done before families will see the fruits of this research in the clinic, but there are clear signs pointing to some promising new opportunities.
Featured faculty
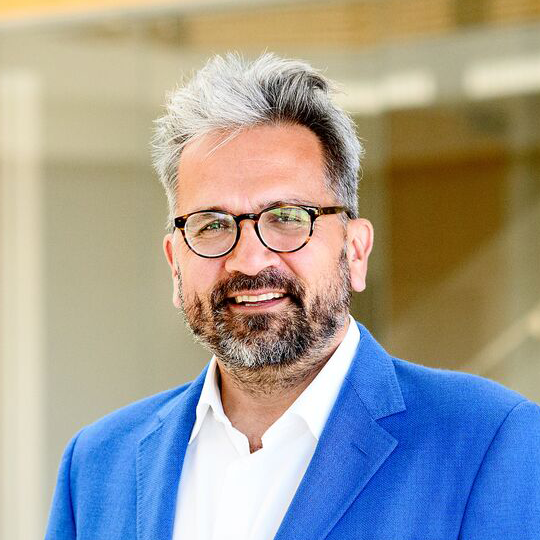
Nevan Krogan, PhD
Director of the Quantitative Biosciences Institute
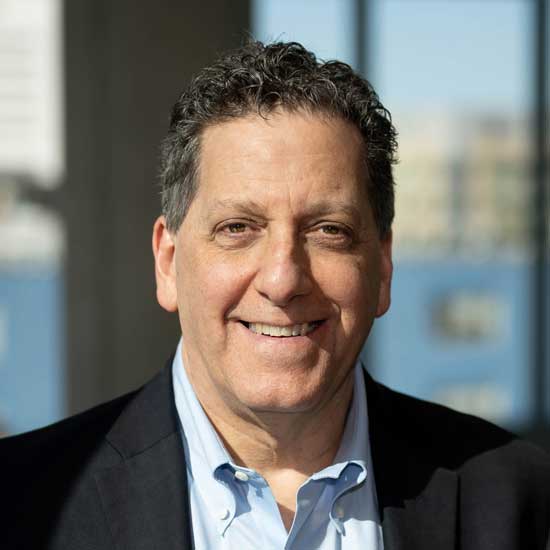
Matthew State, MD, PhD
Chair of the Department of Psychiatry
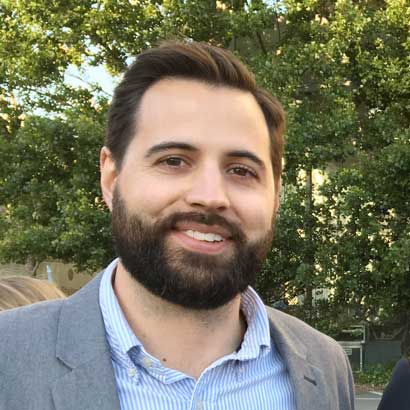
Jeremy Willsey, PhD
Adjunct Professor, Department of Psychiatry
Why has autism been so difficult to understand and address?
Matthew State: A little over a decade ago, my lab and several others were the first to systematically identify genes that carry very large effects for ASD. There was a tremendous amount of excitement about what those discoveries would mean, particularly for children with severe autism and related neurodevelopmental challenges.
Now the field has identified 255 of these genes. But we’ve found that it’s like having a long list of words that are related to some forms of ASD but without the ability to understand specifically how they go together so we can make sense of what causes the syndrome.
Jeremy Willsey: While a long list of genes that impart large biological effects is helpful, several things have made developing treatments from this knowledge particularly difficult. The first is that we’re dealing with the brain, which is the most complex organ in the human body. Second, ASD emerges very early in brain development, making it challenging to study the impact of ASD-related mutations. Third, any single gene can play many roles, particularly in different cells and at different times in the developing brain. So, when we started pulling on the threads of information these genes provided, it was like we were trying to unravel all the biology that unfolds as the brain is taking shape. It was clear that we needed to find some common points across this large number of genes, and working with Nevan we were able to do that.
State: By looking at the protein interactions, we can begin to elaborate the grammar and syntax of this biology. We can see sentence fragments and how pieces might potentially fit together and this promises to give us new ways to think about developing treatments.
What is this new approach and how does it provide that kind of detail?
Nevan Krogan: What Matt and his team have had is a list of genes and mutations. That can only take us so far. What we really need is to understand the biological consequences of each of those mutations. That’s where looking at proteins comes in.
We wanted to look at the impact of mutations in around 100 of the genes that are associated with autism. First, we wanted to know about the proteins these genes code for and what their activity is in a neurotypical situation. That led us to identify about 1,000 additional proteins that we think are somehow connected to ASD. We mapped out how all those proteins interact, essentially the way they talk to each other, when there aren’t any mutations present.
Next, we introduced a large number of mutations individually to see whether it affected how these proteins talk to each other, and we used that information to redraw the map to see the impact of each mutation.
How did you confirm that these mutations worked like you expected in a developing human brain?
Krogan: We used artificial intelligence here to help us interpret these protein-protein interaction maps, something that hasn’t been done before. That was done by a company called Rezo Therapeutics, which has a partnership with UCSF. Using their AI allowed us to narrow in on specific mutations that were the most important to study.
To look closely at the impact of one of these mutations, we introduced it into human stem cells using CRISPR, in collaboration with Tom Nowakowski, PhD, an associate professor of neurological surgery. Then we used those cells to grow what we call organoids, which are tiny bundles of cells that we can use to model what’s happening in a whole organ, in this case, the brain. Again, we saw defects in how the neurons were developing.
So, we were able to go from A to Z, from a set of genes connected to autism to being able to actually see, in this mini organ made of human cells, the biological effect a single mutation, and I think it could be done for many conditions beyond neuropsychiatric disorders too.
Wow, that’s amazing. But how does this new technology get us closer to treatments?
State: Nevan’s technology gives us a completely new, high-resolution picture of exactly what’s going on in the interactions between proteins, including when ASD genes are mutated. That begins to give us the ability to look beyond the original gene to identify interacting proteins we might target to correct the problem, and, potentially, to identify what kinds of molecules or drugs might do that job.
From the standpoint of autism, this approach promises to give us the kinds of opportunities that genetic discoveries are now offering in developing rational therapies for diseases like cancer.
Going back to the language metaphor – we can start to elaborate the grammar and syntax of the biology that underlies ASD. We can start to put phrases together in a way that’s not possible just from looking at the genetics. Where we’ve just had a list of words, we now have some legible sentences, and we can begin to see how those sentences connect with each other.
Willsey: In addition, this approach holds a lot of potential for treatments that can be personalized without having to develop specific therapies for each patient.
Because the genetics of autism and the symptoms associated with it are so varied, the field has been concerned that potential treatments would have to be tailored to small groups of people. A big advantage of focusing on the proteins involved means that we can identify much larger groups where everyone may benefit from the same therapy, which could make clinical trials as well as treatment more effective.
How has it helped to partner with QBI?
State: When Nevan and I met, it was immediately like peanut butter and chocolate. It was obvious that he was developing an extraordinary platform, and that the genes we had discovered carrying large risks for autism could be a great exemplar of how that platform could transform our understanding.
This collaboration is, in my view, at the core of what UCSF does best. With our Psychiatric Cell Mapping Initiative, QBI, the UCSF Weill Institute for Neurosciences, and many cross-departmental collaborations, we’ve been deliberately breaking down the barriers that tend to isolate psychiatry from other clinical and basic science departments at many other institutions. That is what makes UCSF such a gratifying place to be doing this work.
Where do you go from here?
State: A big question of course is whether what we are learning, regardless of how fascinating it is, can be turned into new and better treatments. We believe this work opens the door to a much broader range of opportunities to do just that for the 20% of individuals with ASD who carry the kinds of mutations we studied and who are more likely to have severe autism, epilepsy and markedly delayed development.
A broader question is whether this will ultimately give us insight into the biology of other forms of autism that aren’t associated with these rare mutations or additional developmental problems, and right now, that's an open question.