Superfast Brain Circuit Slams the Brakes on Movement
A new UC San Francisco study of patients with Parkinson’s disease has revealed a pathway that transmits signals very rapidly between two parts of the human brain to govern the complex act of halting a motion once it’s been initiated. The work could potentially help patients with movement disorders or a lack of impulse control.
“This is more than just being able to quickly stop your step into the street if you see oncoming traffic,” said UCSF Neuroscience Graduate Program student Witney Chen, first author of the study published March 9 in Neuron. “This pathway is critical to controlling movement overall. We’re interested in understanding how the brain controls the ways we can stop movement because when this control isn’t functioning properly, it can result in movement disorders such as Parkinson’s, or psychiatric conditions like obsessive-compulsive disorder.”
Though indirect evidence from functional imaging has suggested that such a circuit is present in humans, the new study is the first to confirm its existence and shows that the pathway rapidly and directly conveys signals between a region known as the subthalamic nucleus (STN), which is involved in a number of complex motor and non-motor functions, and an area of the prefrontal called the inferior frontal gyrus (IFG).
“This work is at the interface of cognitive and classic motor systems neuroscience,” said senior author Philip Starr, MD, PhD, the Dolores Cakebread Professor of Neurological Surgery and co-director of the UCSF Surgical Movement Disorders Center. “Stopping movements often involves complex cognition, assessing risk in the environment and risk-benefit tradeoffs. So, this is an interesting system in that it doesn’t directly involve the motor cortex but rather parts of the prefrontal cortex involved in cognition.”
Gathering Information With Electrodes
This study, which involved 21 patients as research participants, was the first to use electrodes placed directly in the brain to gather information about this pathway. The study involved two experiments: In one, the researchers directly stimulated the STN in patients with Parkinson’s disease and recorded the associated response in the IFG, establishing the existence of the pathway; in the other, patients performed a task that involved stopping movement while researchers recorded brain activity in the two regions, demonstrating the pathway’s behavioral function.
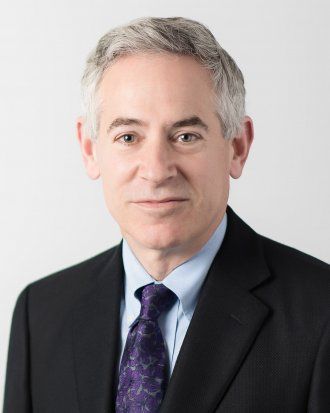
Philip Starr, MD, PhD
The STN and IFG are about 5 cm apart in the human brain, and the response to STN stimulation was detected very quickly in the IFG. These experiments provide the first physiological evidence of a “hyperdirect” pathway connecting the IGF and STN in humans.
In the second experiment, patients were presented with right or left arrows on a screen, which served as a “go” signal to push either a right or left button. At random times they received a “stop” signal within a few hundred milliseconds of receiving the “go” signal. The researchers found that the more synchronized the IFG and STN signals were – the longer both brain regions were simultaneously activated – the faster the subject stopped their action.
Unique Challenges, Opportunities
Recruitment for the study presented unique challenges and opportunities. “These experiments can really only be done well with invasive electrodes at both ends of the pathway,” said Starr, pointing out that implanting electrodes in the brains of human study subjects isn’t something that most researchers can do.
The people who participated in the study were all Parkinson’s patients already slated to have electrodes implanted in the STN region of the brain, a standard procedure for the deep brain stimulation (DBS) treatment often used on patients with mid-stage disease. Because there are no pain receptors in the brain, patients are kept awake during the surgery so that clinicians can confirm placement and function of the DBS device. Thus, the subjects were able to interact with researchers when asked to perform the stop-signal task.
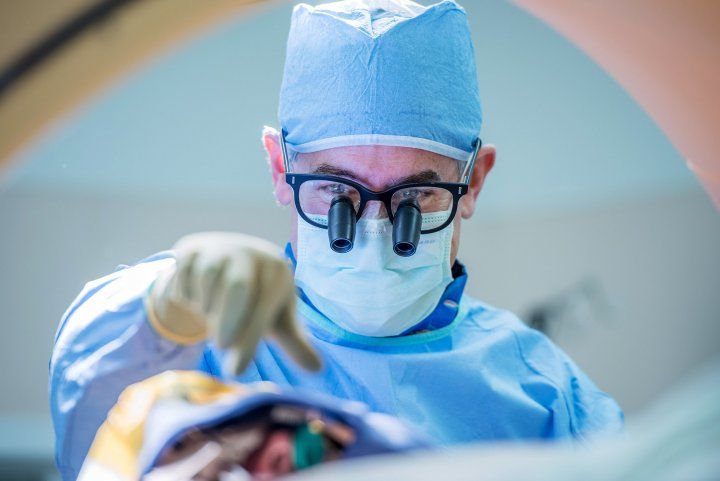
Philip Starr, MD, PhD, performs brain surgery at the UCSF Helen Diller Medical Center at Parnassus Heights. Photo by Barbara Ries
“It's a wonderful opportunity to study the human brain as an intact system, said Starr, a member of the UCSF Weill Institute for Neurosciences. “And fortunately, Parkinson’s patients are especially eager to volunteer. They’re often people who had normal lives for a long time, and now they have this disorder and they really want to contribute to understanding and treating it.”
Chen noted that not only do these experiments take advantage of electrode implant procedures that were already planned, but they don’t require any other penetrations in the brain. In addition to the clinical DBS electrode that was positioned in the STN, a flat array of electrodes that was placed on the surface of the brain could be easily removed after the experiments.
The researchers say their next step is to go a step beyond the stop-signal task and study the role of this pathway in more real-life settings, which they expect to be able to do by gathering data with electrodes that can record brain activity over long periods of time after patients have left the hospital.
“Using this technology, we can start to tease apart what this circuit is doing in real life when people are moving, talking, walking, playing music or sports or whatever they want to do,” said Chen. “We want to know whether we can stimulate this circuit in a certain way to improve gait for a Parkinson’s patient, or inhibition in impulse control disorders. We’re really pursuing these therapeutic aspects, because we think modulation in this circuit can translate to better clinical outcomes.”
Authors: In addition to Chen and Starr, authors included UCSF’s Coralie de Hemptinne, PhD; Simon J. Little, PhD; and Daniel A. Lim, MD, PhD; Andrew M. Miller of the University of Kansas School of Medicine; Michael Leibbrand, of Doculayer Inc., in The Netherlands; and Paul S. Larson, MD, of the UCSF-affiliated San Francisco VA Health Care System.
Funding: This work was supported by the National Institutes of Health (F31 NS103410-01A1).
Disclosures: Paul Larson receives honoraria from Medtronic.
The University of California, San Francisco (UCSF) is exclusively focused on the health sciences and is dedicated to promoting health worldwide through advanced biomedical research, graduate-level education in the life sciences and health professions, and excellence in patient care. UCSF Health, which serves as UCSF’s primary academic medical center, includes top-ranked specialty hospitals and other clinical programs, and has affiliations throughout the Bay Area.