Lyme Disease Ticks Produce Antibiotic That Protects Them From Human Skin Bacteria
Gene Provides Protection During the ‘Really Risky Sport’ of Feeding on Blood
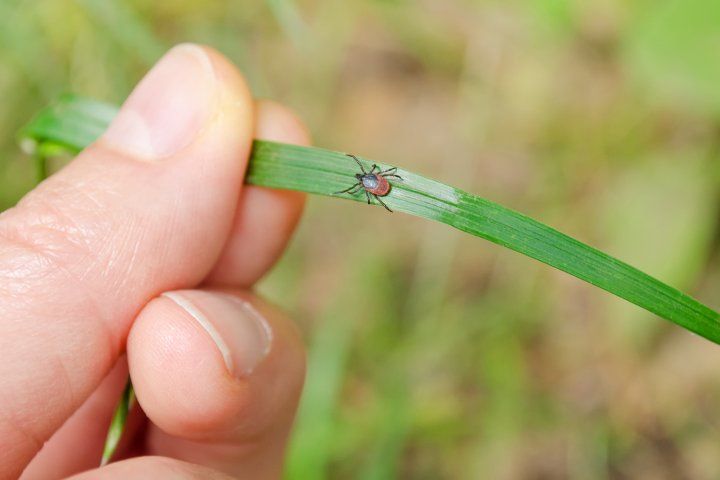
Ticks live dangerous lives, spending most of their time questing for a host across wildly different habitats and seasons. Once they encounter a reptile, bird, or a mammal like us, they become intimately connected with it – and all of its bacteria and viruses – for days on end. Though ticks are notorious for transmitting pathogens such as the Lyme disease bacterium, how does their immune system keep them safe from contracting pathogens themselves?
In a study published in Cell on Dec. 10, 2020, a research team led by UC San Francisco’s Seemay Chou, PhD, provides an answer to this mystery. The work, Chou said, reveals that ticks are exquisitely constructed blood-sucking machines, with immune systems specially tailored for this unique lifestyle. Their defense strategies are carried out both inside and outside their bodies, she said, killing even our resident microbes as they feed on us.
Five years ago, in work published in Nature, Chou and colleagues found a gene in tick DNA that produces a microbe-killing protein. In the new study, senior author Chou leveraged that discovery to show that, without the protection offered by this gene, ticks are vulnerable to infection with Staphylococcus, one of the most common types of “commensal” bacteria. These bacterial species carpet our skin surface, but generally don’t harm us.
“This is the first time anybody’s identified a natural pathogen of ticks, and established a mechanism for it,” said Chou, an assistant professor of biochemistry and biophysics at UCSF and Chan Zuckerberg Biohub Investigator whose work is supported by a Sanghvi-Agarwal Innovator Award. “Ticks pass more microbes to humans, livestock, and other animals than any other known arthropod, but now their own vulnerabilities are on the table.”
The tick gene in question, known as dae2, originally evolved in bacteria, where the protein it encoded worked as an offensive agent against other bacteria. Several hundred million years ago, right around the time that the ancestors of some of today’s ticks began feeding on blood, those ticks “stole” the gene, making it a part of their own genomes.
According to Chou, dae2 represents a rare example of so-called horizontal transfer of a gene from a bacterium to an animal, and the fact that this transfer occurred as blood feeding evolved might not be a coincidence.
“I’ve always wondered why blood-feeding is even a thing,” said Chou. Not only does blood take a lot of energy to process into useful food, but biting on and attaching to much larger animals “seems inherently like a really risky sport.” With a strong, dae2-enhanced immune system, she said, tick species could have flourished, expanding to fill their bloody ecological niche.
When she first began working with dae2 in Ixodes scapularis, the deer tick, Chou thought ticks’ acquisition of the gene must have something to do protection against tick-dwelling bacteria like B. burgdorferi, which causes Lyme disease in humans and other animals. In experiment after experiment, she and members of her lab tried to find a mechanism for the gene to inhibit this bacterium, until they had an epiphany.
It makes no sense for ticks to have acquired this immune effector to kill off the bacteria that it’s most notoriously known to stably associate with,” she said. So instead, the lab began the much more complicated process of looking for bacteria that ticks are not known to live with peaceably. When members of the lab, including co-first authors Beth M. Hayes, PhD, and Atanas D. Radkov, PhD, proposed exploring the idea that dae2 might protect against Staphylococcus bacteria, “I actually pooh-poohed the idea – I bet a beer against it,” said Chou.
But after the dae2 protein was introduced to a cloudy vial of cultured staph bacteria, Chou was shocked. “The tube went from murky to clear in like, a second,” she said. “I was kind of glad to have lost this bet. After two years of trying to figure out what was going on, it all started falling together.”
Now the team had a direction. As detailed in the Cell paper, the researchers embarked on a series of wide-ranging experiments, first comparing dae genes in a range of tick species with the bacterial tae genes from which they were originally derived. With these comparisons and high-resolution protein structures in hand, they used computer models of these proteins to compare their shape and orientation when they came in contact with molecules found in bacterial cell walls.
Next, they moved on to testing the proteins directly against actual molecules extracted from bacterial cell walls. While dae2 protein could quickly degrade this material, taken from common skin bacteria, Tae2 could not. Dae2 also killed a wide a range of bacteria, notably three very common species that are symbiotic partners of human skin.
The researchers then investigated whether dae2 could reach our skin. They found dae2 protein in tick salivary glands and saliva, and observed that, from there, the protein was transferred to the ticks’ blood meal hosts.
When Chou and the team cancelled out the effects of dae2 in a group of blood-fed ticks, using a technique known as RNA interference in some mice and immunizing other mice to the protein, they found higher levels of Staphylococcus bacteria than in ticks with the functioning protein. These ticks also stayed smaller and gained less weight than ticks with dae2.
“This is a new way of thinking about how ticks interact with microbes,” said Chou. Microbes borne by ticks cause disease in humans and animals worldwide, but that’s only half the story, she said. “Their commensal is our pathogen, and our commensal is their pathogen.”
Authors: Joining Chou, Hayes, and Radkov were Fauna Yarza, Sebastian Flores, Jungyun Kim, Ziyi Zhao, and Victoria Bowcut, all of UCSF; Katrina W. Lexa, of Denali Therapeutics, Liron Marnin and Joao H.F. Pedra of the University of Maryland School of Medicine; and Jacob Biboy and Waldemar Vollmer of Newcastle University.
Funding: The research was funded in part by grants from the NIH (R01AI132851, R01AI134696, R01AI116523), Research Councils UK (EP/T002778/1), the UCSF Program for Breakthrough Biomedical Research, and the Sandler Foundation. Additional support came from the Chan Zuckerberg Biohub, the Johnson & Johnson WiSTEM2D Award, the Pew Biomedical Research Foundation, and the Sangvhi-Agarwal Innovation Award. Yarza was supported by the National Science Foundation (1650113) and a grant to UCSF from the Howard Hughes Medical Institute through the James H. Gilliam Fellowships for Advanced Study program.
Disclosures: The authors declare no competing interests.
The University of California, San Francisco (UCSF) is exclusively focused on the health sciences and is dedicated to promoting health worldwide through advanced biomedical research, graduate-level education in the life sciences and health professions, and excellence in patient care. UCSF Health, which serves as UCSF’s primary academic medical center, includes top-ranked specialty hospitals and other clinical programs, and has affiliations throughout the Bay Area.