Scientists Unravel Mystery of Rare Genetic Disorder that Causes Intellectual Disability in Females
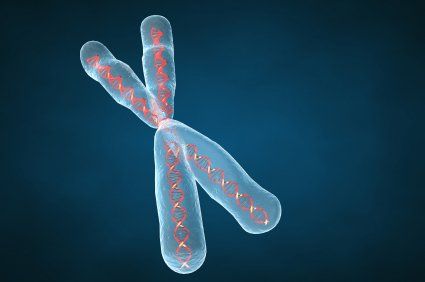
Scientists have made a significant advance toward understanding a rare genetic condition, almost exclusively affecting females, that results in a broad spectrum of neurodevelopmental deficits. Symptoms range from movement and speech impairment on the mild end of the spectrum to severe intellectual disability, autism, brain malformation and drastically reduced brain size on the more extreme end.
DDX3X syndrome, as the condition is now known, was first described in a 2015 study in which scientists sequenced the genomes of thousands of participants and identified an X-chromosome gene called DDX3X that was frequently mutated in young girls diagnosed with unexplained developmental delay or intellectual disability. Though these mutations could account for up to 3 percent of all cases of female intellectual disability, scientists at the time weren’t able to explain how DDX3X mutations might cause neurodevelopmental disorders or why the severity of symptoms varied so drastically among patients.
“If you look back just 10 years, the cause of the vast majority of these cases were unknown. You would see a patient with intellectual disability, autism or developmental delay in the clinic and you wouldn’t know what the molecular mechanism was, you wouldn’t know what the gene was, and you wouldn’t have any insight into how to target the treatment for that specific patient,” said UC San Francisco’s Elliott Sherr, MD, PhD, professor of Neurology, director of UCSF’s Brain Development Research Program and member of the UCSF Weill Institute for Neurosciences.
But now, after conducting the largest study to date of individuals with DDX3X syndrome, scientists have identified specific types of DDX3X mutations that lead to more severe neurological defects. And additional experiments in mouse models of the disorder have allowed researchers to begin to understand how these mutations interfere with brain development on a molecular level. These breakthroughs – a collaborative effort led by Sherr and Duke University’s Debra Silver, PhD – are described in a paper published March 4, 2020, in the journal Neuron, and represent a major advance that may dramatically transform the way doctors diagnose and treat DDX3X syndrome.
The researchers identified 107 patients with mutations in DDX3X who had one or more of the broad spectrum of symptoms commonly associated with DDX3X syndrome – intellectual disability, autism, speech deficits, epilepsy, malformed brain furrows (polymicrogyria), reduced brain size (microcephaly), and other neurodevelopmental deficits.
When they sequenced and analyzed the DDX3X mutations in these patients, they found that the mutations came in a number of varieties – so-called missense mutations change one of the amino acid building blocks in the final DDX3X protein; nonsense mutations cause the translation from gene to protein to terminate prematurely; frameshift mutations change the final protein to a completely different one, due to the insertion of genetic material which alters how the gene is translated into protein; and splicing variants, which alter the way the gene’s protein-making instructions in messenger RNA (mRNA) are edited prior to being translated into the final DDX3X protein.
They also found that only one of the two copies of the gene tends to be mutated, resulting in one intact copy of the gene and one mutated copy. However, the researchers demonstrated that one mutated copy is enough to cause the syndrome in females. (For males, who have only one copy of this X-chromosome gene, mutations are often thought to be lethal in utero.)
The researchers categorized these many varieties of mutation into those that cause the protein to completely lose its ability to function versus mutations that interfere with the protein’s normal function. “In some cases with these mutations, you essentially go from having two copies of the gene to having one copy: you lose a copy because the mutation causes that copy to become completely non-functional,” said Sherr. “On the other hand you can have mutations, missense mutations, where swapping one amino acid with another can have severe effects on the proten without completely destroying its function.”
Patients with the mildest forms of DDX3X syndrome tended to have loss-of-function mutations while those with the most severe manifestations of the disorder – patients with polymicrogyria, cerebral palsy, epilepsy and a lack of ability to walk or speak – tended to have missense mutations that alter the function of the DDX3X protein in ways that seem to actively sabotage the remaining good copy, preventing it from functioning normally.
Additional experiments in mouse models of the disorder led by Silver and her team at Duke helped the researchers confirm the link between certain types of DDX3X mutations and more severe symptoms. They were also able to show how losing DDX3X function interferes with normal brain development. Using the CRISPR-Cas9 gene editing system to deplete the developing mouse brain of the gene, the researchers found that the normal DDX3X protein was needed to turn neuronal progenitor cells into mature neurons. Interfering with DDX3X activity also prevented brain cells from arranging themselves into anatomically and physiologically normal brain structures.
In molecular experiments done in collaboration with UCSF’s Stephen Floor, PhD, assistant professor of cell and tissue biology and an expert in human RNA biology, the researchers were able to show exactly how mutated DDX3X was malfunctioning at the molecular level.
The DDX3X protein is an RNA helicase, a type of enzyme that normally functions to unravel tightly wound structures in RNA that serve as “red lights” during the process of translating mRNA into proteins. These red lights work by creating a 3D structure in the RNA that prevents it from being translated into a functional protein. When the DDX3X protein is functioning properly, it unwinds the mRNA, removing the additional structure and allowing the gene to be translated into a functional protein.
“That 3D structure behaves kind of like an on-off switch for protein translation. Normal DDX3X flips the switch on,” said Sherr.
But mutated DDX3X protein results in the partial or complete loss of RNA unwinding, which prevents certain genes from being translated into proteins. The researchers found that loss-of-function mutations resulted in a partial loss of mRNA unwinding, while those that alter the protein’s function, including missense mutations, seemed to sabotage the remaining good copy and lead to a near complete loss of helicase activity. These results show that the severity of the symptoms seen in DDX3X patients seems to mirror the severity of mutation at the molecular level.
“We can now draw a straight line from diminished helicase activity to more severe clinical outcomes,” Sherr said.
The new findings provide a direct link showing how specific genetic mutations in DDX3X alter the protein’s molecular activity in ways that correlate with clinical severity. These findings may potentially help clinicians diagnose DDX3X syndrome earlier and help the parents of children with the disorder plan better for the future. The new findings may also lead to improved treatment options.
“After the first round of DDX3X papers came out, many people started studying the disorder. We’re hoping that our paper will inspire other researchers to jump in,” Sherr said.