Three-Parent Babies: The Science of Replacing Mitochondrial DNA and What Remains Unknown
Mitochondrial replacement therapy (MRT) has now been used in humans to conceive a “three-parent baby” to prevent inherited mitochondrial disorders, but there remain questions about the effectiveness of the process.
Last September, the New Hope Fertility Center in New York City announced the birth of a baby boy in Mexico who was conceived through the therapy. A child conceived this way carries the nuclear DNA from two parents as well as the mitochondrial DNA from a second woman who has effectively donated her healthy mitochondria. The technique – which isn’t approved in the U.S. – can help mothers who have defective mitochondrial DNA to avoid passing on the mutations to their children.
Patrick O’Farrell, PhD, professor of biochemistry and biophysics, studies the biology of the mitochondrial genome. His lab uses fruit flies to study how mitochondrial mutations are inherited and what happens when two mitochondrial genomes compete against each other. He answered some questions about what we know and don’t know about MRT.
What is the mitochondrial genome and what does it control?
Mitochondria originated as an intracellular bacterial infection that adapted over billions of years to become an organelle that is often referred to as the powerhouse of the cell. During evolution, the original complexity of the bacteria was greatly reduced and most of the genes were moved to the nucleus. But the transfer of responsibility was not complete and the mitochondrial genome, in most animals, still retains 37 genes.
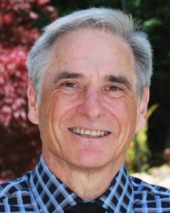
These 37 genes are essential to the electron transport functions that are the chief energy-providing systems for the body. We know what every one of these genes does in the body, but the final outcome of disruptions to these genes, even when you know what they’re supposed to do, is hard to predict because the body has all sort of regulatory loops that adjust to defects and imbalances.
Unlike the nuclear genome, which is a combination of contributions from both your parents’, you inherit only your mother’s mitochondrial genome. We call the mitochondrial genome the “very lonely genome” because your mitochondrial genome will never encounter another person’s mitochondrial genome. The species is divided; every female passes on her mitochondrial genome, and, so long as the female lineage is unbroken, this genome will be passed on, always in isolation. But this lonely genome will accumulate change through mutations. The changes will never be shared, and different changes will accumulate in each female lineage of mitochondrial DNA, so that lineages do diverge over time.
How common are mitochondrial disorders? Would a mother know if she were carrying mutations related to mitochondrial disorders?
The prevalence of maternally inherited mitochondrial disorders is estimated to be roughly 1 in 5,000 adults. Some individuals who carry mutations are relatively healthy, but their children may have a high probability of showing mitochondrial disease—these cases can be very severe with children dying in infancy with wide ranging physical and mental defects.
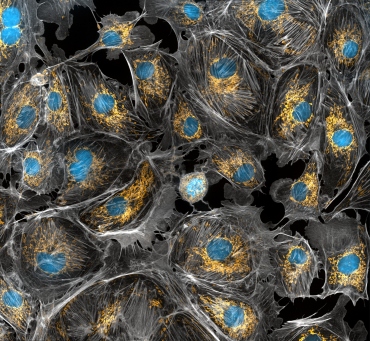
The relationship between mutations and symptoms is complex because cells carry many copies of the mitochondrial genome, typically about 1,000. If a person has some copies containing mutations, they can show a range of symptoms whose severity depends on the proportion of functional and defective genomes that they carry.
A mother may not know that she’s at risk of having a child with mitochondrial disease. The inheritance of mitochondrial genomes does not follow the regimented pattern of nuclear genomes, so a mother that is healthy but carries some mitochondrial disease mutations might pass on a higher proportion of mutant genomes to her child.
How is mitochondrial replacement therapy different from gene editing?
In gene editing you’re actually changing the existing genetic code. Mitochondrial replacement therapy is different because there is no change in the DNA. You are just changing the source of the mitochondrial genome. It’s more a kind of transplantation, where you’re rescuing things, like a heart transplant.
If a woman chooses MRT to have children, you would take the nucleus from one of her egg cells, transfer it to a donor egg cell that has had its nucleus removed. The egg cell can be fertilized before or after. So all the nuclear genes come from the parents and it’s the usual two-parent genetics for everything except the 37 genes carried by the mitochondrial genome.
The intention is that all the mitochondrial DNA comes from the donor, but that turns out to be impossible.
Does this really mean that a child born through MRT would have three parents?
It’s true that the baby has three genetic inputs and I have no particular argument with the term “three-parent baby” except it should be clear that they’re tremendously unequal contributors. It’s 99.9 percent normal genetics.
In gene editing you’re actually changing the existing genetic code. Mitochondrial replacement therapy is different because there is no change in the DNA.
One of the concerns with MRT is that some of the maternal mtDNA will be transferred along with the nucleus into the new egg cell and that two mitochondrial genomes will compete with each other. What do we know about this competition?
The new egg cell will have a mix of mitochondrial genomes, maybe 99 percent from the donor and 1 percent from the mother. So the mutant genome is still there, it hasn’t been eliminated, just reduced dramatically.
In our work using fruit flies, we ask how the genomes compete with each other. You’re putting into one arena competitors that have never seen each other before, and genomes are out for themselves. If a genome has a mutation that makes it replicate faster, it has an advantage and takes over – we call these “bully genomes” and they displace “wimpy genomes.”
Our research shows that bully genomes have changes in the regulatory region that control replication. A very small difference in replication rate is positively selected for and can very quickly eliminate a wimpy genome.
Do the consequences of this competition occur during embryonic development or can they occur later in life?
If there were a real “bully-wimp” mismatch situation, I would strongly expect it to show up very early. There is a huge expansion of population of mitochondria during the production of the baby; as you age there is not as much growth. During this increase in the population of the mitochondria, a bully genome would have the opportunity to out compete the more slowly replicating genome.
One fact that is pretty clear is that mitochondrial DNA is not completely stable and that you’re constantly making new mitochondrial DNA.
But we think some changes may appear later in life, maybe at a more subtle level. One fact that is pretty clear is that mitochondrial DNA is not completely stable and that you’re constantly making new mitochondrial DNA.
I would strongly predict that this child born in Mexico, who is healthy as an infant and has the vast majority of his mitochondrial DNA from the donor, will go on to do very well.
How might MRT fail and what are some ideas to make MRT more successful?
MRT can provide a wonderful benefit to families carrying such mutations, and the only major concern is that an individual application might fail, not that it is a threat to society and human genetics.
In a recent study using human cells in the lab, 13 out of 15 egg cells after nuclear transfer were dominated by donor mitochondrial DNA, and two reverted to the maternal mitochondrial DNA. That might be good enough to satisfy a lot of parents.
There are two reasonable strategies to make MRT better. The first would be to match donor and maternal mitochondrial genomes. If the genomes are well-matched then the procedure is more likely to have a good outcome. Or, make sure the donor’s is always the bully genome – here there will be a mismatch, but the winner is always the donor’s mitochondrial genome. The later strategy would have the advantage of fully eliminating the mutant genome so that it does not re-emerge in later generations.
These strategies depend on knowing more about the biology of mitochondrial genomes. One of the things we’re trying to figure out is why there are bullies and wimps and how to identify the more powerful replicators. What we’re finding is that this might actually depend on the interplay between nuclear and mitochondrial genes.