Lights, Camera, Enzymes!: A Conversation with Chemist and Protein Expert Brian Shoichet
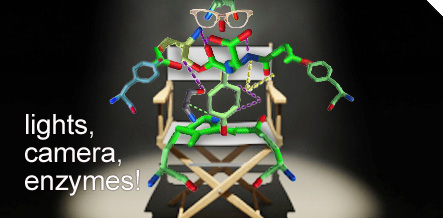
Enzymes really need to hire a publicist. Genes had been hogging the headlines for years until proteins with a capital “P” pushed them aside. Now you can read about big proteins everywhere. But enzymes – those cutups in the protein classroom – have never had their day in the sun. Maybe we can blame it on those biotech companies with the odd monikers. Enzymes sound like a name nobody liked.
That not’s true of pharmaceutical chemist Brian Shoichet, PhD, who last year pushed enzymes into the spotlight at last. His big discovery? Figuring out a way to determine what an enzyme does by how it is shaped.
The model enzyme he used, TM0936, lives in boiling water at the bottom of the Mediterranean. Original software created in Shoichet’s School of Pharmacy lab, which is designed to find patterns in the metabolites, or byproducts, of TM0936 – coupled with computers that are at least 1,000 times faster than those used just two decades ago – soon detected a pattern.
A little more sleuthing and a lot of chemistry later, Shoichet had the proof he needed. TM0936, it seems, has a special affinity for adenosine, a messenger molecule in all forms of life.
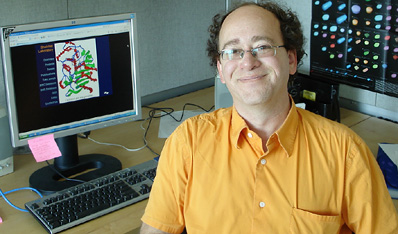
Brian Shoichet, PhD
What does this mean to us? Well, we don’t live near a volcanic ocean vent. So, on the surface, the discovery seems interesting, but hardly a wavemaker.
Better think again. The technological approach developed in Shoichet’s lab could save pharmaceutical companies huge amounts of time and money now spent in following false drug leads suggested by less sophisticated methods. And that approach is a direct benefit to human health.
Apart from that, Shoichet explains with a smile, many theoretical discoveries turn out to have very practical applications – yet another reminder that the best science is often the most unscripted kind.
Alternative content
Related Links
- Scientists Figure Out How an Enzyme Works by Knowing its Atomic Structure
- UCSF News Services, July 3, 2007
- Shoichet Laboratory
Transcript
Jeff Miller: Hello I’m Jeff Miller and welcome to Science Café, today I’m with Brian Shoichet, a professor of pharmaceutical chemistry in the UCSF School of Pharmacy, welcome Brian.
Brian Shoichet: Great to be here.
Miller: I’m going to start off with a big question at the moment: When you look at the state of science in the U.S. today, what do you see are the biggest threats and the biggest opportunities, and how do those play out in your lab?
Shoichet: I’m not expert in that sort of policy area, but the two biggest challenges to science are always funding – we always feel like we’re under funded, and right now it’s particularly acute with what we call the flat funding of the NIH, it hasn’t grown for 5 years, inflation has grown, and science inflation is higher for the average economist, so that’s a big hit, it’s particularly bad for junior scientists just coming into the field. It’s a much harder time now for them than it was for me when I was starting out.
For my lab, we’re sort of set and can muddle through this tough time but for them it’s very hard with sort of our seat corners being eaten.
Miller: I know there’s a lot of talk on the impact of graduate education, does that play out at all in your lab?
Shoichet: We don’t see that effect so strongly, but we’re sort of in a great position here in UCSF because we’re one of the premiere flagship institutions on science, and the problems hit us last. For the second tier, and that’s not meant to be disparaging in any way, institutions, you know I was at North Western for the first six years of my career and they have a tougher time of it than we do.
We’re also starting to feel the pain but they cut us last.
Miller: We’re you the first scientist in your family?
Shoichet: In my immediate family, yes – I almost said no because my sister and I are almost the same age and she’s also a scientist.
Miller: What does she study?
Shoichet: She’s a chemist, a biomaterial chemist.
Miller: Were there other members of your family
Shoichet: No, they’re all business people and lawyers...
Miller: So how did this turn toward science come about?
Shoichet: My father is more of an engineering type, so many that had something to do with it – we had great science teachers in school, I think that was the biggest part of it.
Miller: And you grew up in-
Shoichet: Toronto, Canada.
Miller: Do you get the chance to read a lot?
Shoichet: Yes, a lot of history, when I’m not reading science.
Miller: What kind of history, intellectual history, or-
Shoichet: Well it’s all sort of intellectual history – so now I’m reading a book by Tony Jut called Post War, it’s about Europe after the second world war, that’s very modern history -
Miller: If I remember correctly you were a history major, right?
Shoichet: Yes
Miller: So why the turn towards science?
Shoichet: Well I was at MIT, so (laughter) so the history was just a sidebar for me, I was a chemist.
Miller: So I know you’ve been at UCSF for a number of years
Shoichet: Almost five
Miller: Can you give me a quick explanation of what molecular docking is and why UCSF has a reputation in this area?
Shoichet: Molecular docking is the attempt to fit drug-like molecules, organic molecules into the structures of the biological molecules that are meant to receive them. The idea is you start off with typically a protein, enzyme structure where you know the position of every atom and that’s no joke, because the atoms are very small, and there is among the order of three thousand of them, and they are all connected so there’s a lot of moving pieces, so it’s this very complicated puzzle. And what you’re trying to do is fit the last piece or two into that puzzle, and that last piece or two are the sub-straits or the inhibitors, the things the enzyme will react with or the things that will stop the enzyme from reacting.
Miller: And being able to visualize this was one of the big milestones
Shoichet: Yes. That started to become possible in the mid 1980s, and that was technology that was pioneered here by a guy named Bob Langrigch at UCSF. I became involved in the late ‘80s as a graduate student, actually I was here at UCSF at the time with a guy named Tack Kuntz, and he developed the methods to try and fit the organic molecules, the drug-like molecules into these three thousand atom structures. For a chemist, three thousand molecules is a giant molecule. The molecules, the drugs that we’re trying to fit in were like 20 atoms, much much smaller. But it’s like fitting the last piece of the puzzle – the problem is – the reason you need to do this with computers is that the puzzles, the proteins are so complicated they’re like these molecular canyons, imagine Bryce canyon, and you’re trying to stick a wedge-like rock into Bryce canyon to plug a little bit of it up, that’s about the size scale.
And there are all these different places you can put it.
Miller: Did it take huge advances in computing power to make what is possible today?
Shoichet: Yes (laughter) in computing power and in our understanding of how the pieces fit together.
Miller: So proteins are your bread and butter obviously, give me some idea of the type of research programs you have going on in your lab at the moment.
Shoichet: Mostly we’re interested in technologies around drug discovery, and the idea is you start with the structure of the protein, and proteins are the things that do everything in the body, so from thinking to salivating to digestion, it’s all proteins, and so that’s a good place to start. And drugs mediate their actions by binding to proteins. The things you sallow get digested, they go into the blood stream, and then they all bind to specific protein molecules. So our projects are mostly around developing these technologies to predict what will bind where.
Miller: And what success have you had so far?
Shoichet: We’ve had a lot of success I think driving the technologies and understanding early problems in the discovery – for instance – we’ve had success in starting with the protein structure and coming up with completely new templates that will bind to that structure. And we’ve had success understanding some of the “false hits” that come up in drug discovery in the pharmaceutical industry.
Miller: What is a “false hit” exactly?
Shoichet: A molecule that looks like it’s binding to the protein because it’s inhibiting its action, for instance, but actually is doing something completely different. It is inhibiting it but it’s inhibiting it for some pathological reason like it’s precipitating it.
Miller: So it might actually be causing a problem-
Shoichet: Yes, they’re often very non-specific and – at UCSF a major focus is this computer based method where you’ll take a million molecules, and you’ll screen them using a computer to take each molecule, fly it into this Bryce canyon and predict if it will bind, but in the pharmaceutical industry they’ll literally take a million molecules and experimentally test each one and you think, oh well that seems better than using a computer program because you get the real answer, but it turns out that their answers are clouded by a dense fog of “false hits” and figuring out what is really working and what isn’t is their number one problem, and that’s an area of interest for us too.
Miller: What is the “ah ha!” moment when you know you actually have a lock-up mechanism, something happen on the screen or is there a change in the conformation of the protein in the screen, or how do you even know what you’re looking at?
Shoichet: Simulations are the place where we start but we never end there, the “ah ha!” moment comes at 2 a.m. in the lab when you’re looking at the actual experimental results, and you say oh boy, it really worked. The computer is just a guide to the actual wet chemistry experiment which we also do in our lab.
Miller: But it speeds up the process because you can eliminate a lot of possibilities-
Shoichet: Oh yeah, it’s huge, we wouldn’t get anywhere without the computer simulations
Miller: Can we gauge how much faster things are these days? Is it a hundred times’ faster than it used to be 20 years ago, or have you ever thought about it in those terms?
Shoichet: In terms of computer power, it’s at least a thousand times faster, but on top of that the methods for doing things have also improved. So in that sense maybe you’d say we were ten thousand times better now than we were 20 years ago.
Miller: I want to go back to this “false hits” thing for a second, is it possible that a “false hits” might explain a disease state that was otherwise unknown, because you could actually have identified and negative something that turns into something interesting?
Shoichet: I can’t rule that out, but most of our discoveries have been in the area of weeding out molecules that look like they’re doing something good but in fact are not doing anything useful.
Miller: OK. I know there was a lot of publicity about a paper that was published last summer I believe about enzymes, why don’t you tell me about that?
Shoichet: This is one of the grand ideas in our field, which is if you know what enzyme looks like, you can figure out what it does. And now, these days we have a lot of pictures of enzymes, of these Bryce canyon molecules, and for many of them, we know what they look like but don’t know what they do. So the idea was, could we start with the structure of the enzyme, and from first principles predict what it did, and so we started off with a structure of an enzyme from this bacteria Thermotoga Maritima which is a very arcane little bacteria – well, I don’t know how little it is, it lives on sea vents, hot ocean vents in the Mediterranean. It -
Miller: Only in the Mediterranean?
Shoichet: As far as I know, but it has cousins that live in ocean vents all over the place. There are this class of bacteria called thermophilic or Hyperthermophilic bacteria, that live in basically boiling water at the bottom of the oceans at extremely high pressures, parenthetically is one of those amazing things about molecular evolution, life has spread all over this planet, there is few places where it can’t find a niche, and these bacteria are an example of that.
Anyway, they produce an enzyme which was called TM, for Thermotoga Maritima 0936 – didn’t have a real name because nobody knew what it did and so our goal was to figure out what it did and it was sort of a proof of concept study for us, could we do it for this enzyme where we had really structure, because if we could, maybe we could do it for lots of others.
Miller: And how big a structure are we talking about?
Shoichet: It’s an average type enzyme, I said 3000 atoms, maybe this guy had 2500...
Miller: OK, so you have the atom, you have the structure; you know what you want to do, how did you get to the result that you ultimately did?
Shoichet: We said, well let’s assume that this enzyme works on metabolites, those are things in the body and in this case the bacterium that the bacteria has to deal with and to convert foreign sources of molecules into food or energy or things it can build with. And we assume it will interact with one of those and there were about 4000 of those, maybe more.
And then we said, well, let’s take each one of them and fly them into the site of this protein and figure out which one does it recognize.
Miller: And how long did it take you before you found the recognition pattern?
Shoichet: The calculation in itself is pretty quick, a couple of days…
Miller: Only a couple of days-
Shoichet: But we had to do it multiple times because no one had ever done this before and we were feeling our way and so we would sort of boot strap our way until the patterned emerged, and we had to invent the technology to do it, there were differences in what we normally do, technically.
Miller: What type of technology did you need to create?
Shoichet: We had to… Enzymes catalyze reactions. Usually we’re interested in a drug that will be the last puzzle piece that will fit and stop the enzyme from working. Enzymes have to break molecules apart or put them together and that involves chemical reactions and real changes in the structure, so it’s like breaking the puzzle piece in two, for instance. And to do that we have to change how we modeled molecular flexibility and the basic structure. We had to predict what the reaction would actually be.
Miller: Is this a software coding-
Shoichet: Yes.
Miller: So it’s all programming, you have people in your lab that do this...
Shoichet: Yes, we have a very talented post-doctoral fellow named Johannes Herman, who has now gone on to work at Rosch Pharmaceuticals.
Miller: So here we are, so you’re testing this out, you have results in a couple of days, and you keep testing to make sure there’s a pattern that emerges, and then what?
Shoichet: What was really exciting was a pattern did emerge. All the molecules that scored best by this method, were derivatives of adenosine, and adenosine is a well known molecule that is used as a messenger in all forms of life, and it’s also a precursor to one of the DNA bases, adenosine monophosphate, so it’s a really essential molecule in all forms of life from bacteria to humans and everything in-between. And what we found was adenosine and all sorts of variations of adenosine that are used in metabolism scored at the top of the list, and what this told us was, according to the docking program, it’s the derivative adenosine that’s being recognized, and not only that, they were all recognized the same way, they were all undergoing exactly the same reaction, it’s called the de-animation reaction where nitrogen is hydrolyzed by an incoming water and leaves and replaced by an oxygen. And that would produce a second, very well known metabolite called anisine.
And based on that we thought maybe it was adenosine, so we tested that with our collaborators in Texas at Frank Brushell’s lab and it turned out to be true. .
Miller: So what does this tell us now?
Shoichet: It tells us that this enzyme works on adenosine, and what it also told us, which we didn’t know before was that this bacteria has a whole set of enzymes around this reaction that create the precursors for this reaction, and take the products of this reaction on, so it’s what you call a metabolic pathway. We knew about some of the other enzymes but we didn’t know they were connected with this one. And once we knew what this guy did the scales sort of fell from our eyes and we could see that there was an entire metabolic pathway in Thermotoga Maritimas, who cares, devoted to these transformations on Adenasine.
Well it turns out that this pathway seems like it exists in at least 78 other bacteria as well and probably in many others so it looks like it’s a conserved pathway for converting metabolic molecules from one state to another, and at least it’s wide spread in bacteria.
Miller: So when we talked at the beginning about drug discovery, how does this relate to new and better drugs, just in theory?
Shoichet: Well it’s the technology that matters, I mean Thermotoga Maritima is not a pathogen, it lives at 80 degrees centigrade so I don’t know what that is in Fahrenheit but extremely hot, and doesn’t live in humans so it’s not a threat to us or livestock or anything. But the method could be quite useful for predicting substrates and for predicting what you call receptor agonist, things that turn receptors on and off. Because it’s one of the really early studies that show that you can capture not only how to inhibit an enzyme but figure out what it does.
So how would it be used in drug discovery? It’s the discovery of other things like that for enzymes that are pharmaceutically interesting and relevant.
Miller: We often hear that the basic science like this-- you’ve made this fundamental discovery about bacteria – but it doesn’t necessarily translate immediately to human benefit, and there’s a lot of money invested in it, so what do you say to people who question whether or not this really has any long term value, that it’s really an indulgence to have scientists continue to explore in this fashion when there’s really not guarantee there will be any pay off at the end?
Shoichet: Well, that’s for sure true, there is no guarantee for any given scientific research program, but overall the scientific enterprise has proved itself over and over again and I think when you look at life saving drugs and the changes in medical technology upon which we all depend, those have all come from basic research, and it’s expensive because a lot of it turns out to be interesting and yet there’s no immediate practical relevance that can be seen, but every field of science builds on this massive body of knowledge and things work out in strange ways, things that seem very theoretical turn out to have a practical benefit down the road.
So when people say, well really, shouldn’t we be spending our money on feeding the poor – I think we should be spending our money on feeding the poor, but for our heavily technological society, investment in science, seems to me, is completely critical. And I guess it’s what they used to say about the space program, you don’t know exactly which result is going to pay off, and it would be unwise to try and script that too heavily, because we’re supposed to discover new things, who knows how a new thing will play out? But what we can say is there’s a desperate need for new things, to make our lives better and those of our children.
Miller: Last question, what’s next for you on your research agenda, are you going to continue with this study, or are there new things in the works?
Shoichet: Yes, yes we’re still super excited with this result; you know it came out in a journal called Nature which among scientists is the “big” journal, so we’re excited by that reception.
One of the other really exciting things that were dealing with right now is predicting side effects and off target effects for drugs – there’s this saying, “show me a drug that doesn’t have side effects and I’ll show you a drug that doesn’t have any effects whatsoever” – so what we’re trying to do now is computationally predict what the side effects will be, and also what we can exploit.
It turns out that a lot of drugs that have been discovered were – this is an example of the unpredictability of science – a lot of the drugs that have had a huge effect were developed for one thing, and it turns out that they had an application in a completely different field because of some side effect, which turned out to be a really interesting and useful side effect, we’re trying to predict those. So that’s an exciting area for us.
Miller: That would be very exciting. Brian, thank you so much for joining us on Science Café.
Shoichet: My pleasure, thanks for having me.