Biology's Next Big Bang, Part 2 of 2
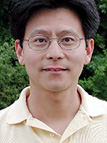
Chao Tang
In the first installment of my conversation with Chao Tang, PhD, a professor in the UCSF School of Pharmacy's Department of Biopharmaceutical Sciences, as well as a QB3 faculty affiliate, we discussed how biological systems are a "talking" community and that the emerging field of systems biology seeks to uncover not only who talks to whom, but how the messages are being communicated and how they mobilize the community into action. Moreover, Tang asserts, biology is on the verge of an intellectual explosion similar to that which occurred within astronomy in the 1600s, when the laws of planetary motion and gravity were postulated and proved.
Scientific revolutions, of course, do not begin at the barricades, but at the lab benches and computer stations. And it is there that Tang's work has demonstrated a simple, but powerful truth.
Evolution, it seems, has put a priority on exactitude and dynamism, often in the same system.
"The cells that make up a system operate in a dense, dynamic and fluctuating environment," says Tang. To make sense of how cells work requires that Tang ask three fundamental questions. What is responsible for robust function, that interplay of hard wiring and dynamism (a folding chain of proteins comes to mind)? Or, to approach the matter differently, if Tang were starting from scratch, how would he wire a system to produce these kinds of fixed and fluid properties? And how would he then test his assumptions experimentally?
This is the region where mathematics reigns, where nonlinear dynamic systems theory and its various equations attempt to map out and make sense of the fluctuating — some might say chaotic — business of a living organism. In some ways, it is akin to observing all the moving parts of an urban transportation system, including its passengers, assigning numerical values to everything, then selectively shutting down different routes, or following subsets of passengers throughout the day and altering your equations based on the results. That's not to mention keeping track of other intersecting system variables such as the weather, taxi strikes and changing gasoline supplies.
"There's always this back-and-forth between your predictions and your data, which we use to improve our models," Tang explains. The changes spark new questions, new thoughts and new experiments. "Why is it wired this way? What can I perturb the next time? What are the potential drug targets? And did the results shed light on other systems? It's a coevolutionary process, " he emphasizes.
It is also a long-range one. Some of Tang's modelers and experimentalists are based at Peking University, necessitating a steady stream of almost daily emails from September through May during the UCSF academic year, when he is based in San Francisco.
The physical distance and the demanding nature of the science could be a recipe for confusion. But Tang has hit upon his own simple and powerful truth. "You learn the most when you learn how to simplify complicated, multi-level diagrams to isolate the key elements of a system," he says. A case in point is yeast, or more precisely the cell cycle regulation system in yeast, which ensures that yeast buds and divides.
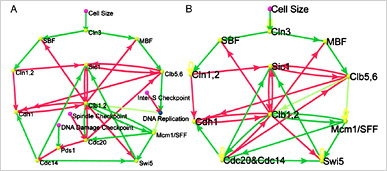
(A) The cell-cycle network of the budding yeast. (B) Simplified cell-cycle network with only one checkpoint "cell size." (See larger)
About 800 of a yeast cell's 6,000 genes are involved its cell cycle. Tang and his team first narrowed the list of key regulators to about a dozen, and have since trimmed this list to three regulatory modules that, as he explains, "capture the essence." In doing so, he also discovered a mathematical meaning for the checkpoints, which yeast has incorporated into its division process to ensure order. If there is the slightest misstep, a red light comes on and the whole system is lured to a rest stop until things are in order again. "That's why the yeast cell cycle rarely makes mistakes," he adds.
Math and engineering are not typically the stuff of fancy. But Tang has a way of describing his work that makes it all seem, well, fun. Perhaps it is because he is a physicist who found his way to biology through play at the NEC Research Institute, based at Princeton. When I say "play," I'm not talking sports or cards here, but the kind of open-ended freethinking (the opposite of daydreaming) that encourages ideas, connections and insights to bubble up into the conscious mind.
"For the 10 years I worked there [1991 to 2001], I was never asked what I did or pressured about grants or committees. In fact, there was no pressure at all," Tang recalls with some wistfulness. The goal of the institute was to mix physicists and computer scientists together and let them develop ideas that would eventually affect human life in a positive way. These ideas included switching research gears, if called to do so by your curiosity — a feat Tang successfully completed in the late 1990s after a six-month brain diet of literature and science meetings outside his discipline. "It was during that time I read Ken Dill's paper on protein folding and it suddenly all sank in. I wanted to work on biological problems."
At UCSF, he has done precisely that, and if his quest ends successfully, it might be rooted in the more holistic approach to problem solving in his native country, which, historically at least, favors systematic insight over reductionism. "In Chinese philosophy, we try to understand the whole, to infer from the top down, which is a good way to approach systems biology. Chinese medicine doesn't have parts, but eventually we hope to understand biological systems from their parts — a case of East meets West."
Alternative content
Related Links
- Tang Lab
- -
- Peking University Center for Theoretical Biology
- -
- The yeast cell-cycle network is robustly designed [abstract]
- Proceedings of the National Academy of Sciences 101;14:4781-4786
- NEC Labs America [formerly NEC Research Institute]
- -
- Systems Biology: A User's Guide
- Nature, November 2006